Introduction
Among the Fusarium fungi genus there are certain species that, due to their prevalence and potential mycotoxic effects, have a significant importance. It is important to highlight Fusarium verticillioides and Fusarium proliferatum since they are widely distributed in various crops, with a special impact on corn production worldwide. Their ability to infect different plant structures, such as roots, stems and cobs, compromises the quality and safety of the grains. These fungi species are producers of several mycotoxins, including Fumonisins, which are associated with adverse effects on animal and human health (Gai et al., 2018). The synthesis of these toxins is regulated by a group of genes located at the FUM locus, which participate in complex networks of signaling and genetic regulation. In addition to their role in feed contamination, fumonisins have been identified as virulence factors, as they influence the fungus’s ability to colonize plant tissues. Research into the regulatory mechanisms of these toxins and their interaction with the plant defense response is crucial to develop control strategies that minimize their impact on agricultural production and food security.
Pathogen colonization routes
Fusarium is a field fungus whose proliferation and production of mycotoxins occurs mainly in the crop environment. Colonization by Fusarium species can occur through multiple routes of entry, and these various strategies are key to its success in tissue colonization and ensuring its survival, which severely affects various crops, such as maize (De la Torre-Hernández et al., 2014).
The main routes of infection are:
- Systemic infection in seedlings: The fungus can infect seedlings from germination, penetrating directly into the seed or through the roots. Although initial colonization is mostly limited to the roots and mesocotyl, the fungus can advance to the stem, albeit in a limited way.
- Infection through stigma: The most common route of infection of the cob occurs when spores of the fungus, carried by air or rain, are deposited on the stigma. From there, the fungus can grow and reach the grains, even in the absence of mechanical damage.
- Infection by mechanical damage: Corn pest insects, when feeding, can damage the plant’s tissues, creating entry points for the fungus. In addition, some insects act as vectors, transporting fungal spores from one plant to another.
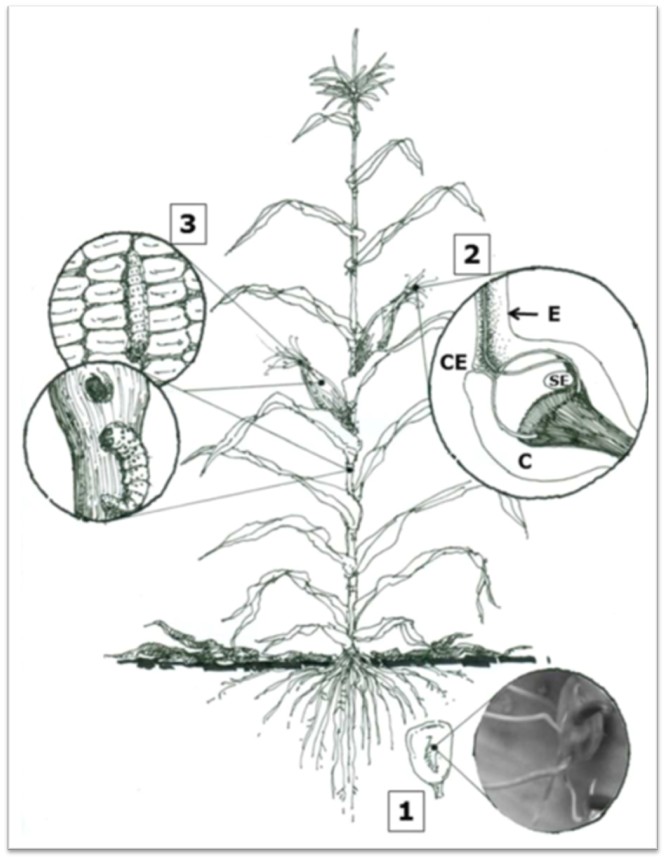
Figure 1. Different routes of entry of Fusarium verticillioides to the corn plant. 1. Systemic infection of seedlings 2. Infection through stigma. 3. Infection through mechanical damage (De la Torre-Hernández et al., 2014).
The ability of Fusarium verticillioides to use multiple routes of infection (figure 1), together with the support of insect vectors, contributes to its high incidence and severity in crops. Understanding these infection pathways is crucial to developing effective control strategies and protecting maize production.
Metabolic pathways and Fumonisins synthesis
The biosynthesis of fumonisins by Fusarium verticillioides is determined by the gene sequence of the FUM locus (figure 2). As shown in figure 3, this process begins with the formation of an 18-carbon polyketide linear chain, which constitutes the base structure of fumonisins. This synthesis is carried out from acetyl-CoA, malonyl-CoA and S-adenosyl methionine, through the action of the enzyme FUM1 (Proctor et al., 1997).
Subsequently, the FUM8 gene, encoding a α-oxoamine synthase, catalyzes the condensation of the polyketide chain through the decarboxylation of amino acids and acyl-CoA thioesters. In this stage, two additional carbon atoms from alanine are incorporated, generating a 22-carbon compound called 2-amino-12,16-dimethylenecosane-3-one (Seo et al., 2001).
The enzyme FUM13, a short-chain dehydrogenase, reduces 2-amino-3-oxo-12,16-dimethyliscose (a ketone intermediate) to 2-amino-3-hydroxy-12,16-dimethylylicosane (Yi et al., 2005). In addition, the enzymes FUM2 and FUM6 catalyze hydroxylation at the C-10, C-14, and C-15 positions (Desjardins et al., 1996).
In the final phase of fumonisin biosynthesis, the FUM11 transporter facilitates the transfer of tricarboxylic acid precursors from the mitochondria. Finally, the enzymes FUM7 and FUM10 convert these precursors into an acetyl group, thus completing the biosynthesis of fumonisins. (Kolawole et al., 2021)

Figure 2. Biosynthetic fumonisin genes in Fusarium verticillioides (FUM locus). The gene that regulates the group is indicated by the golden arrow. Genes not necessary for fumonisin biosynthesis are indicated by the black arrows. .(Butchko et al., 2006; Seo et al., 2001)
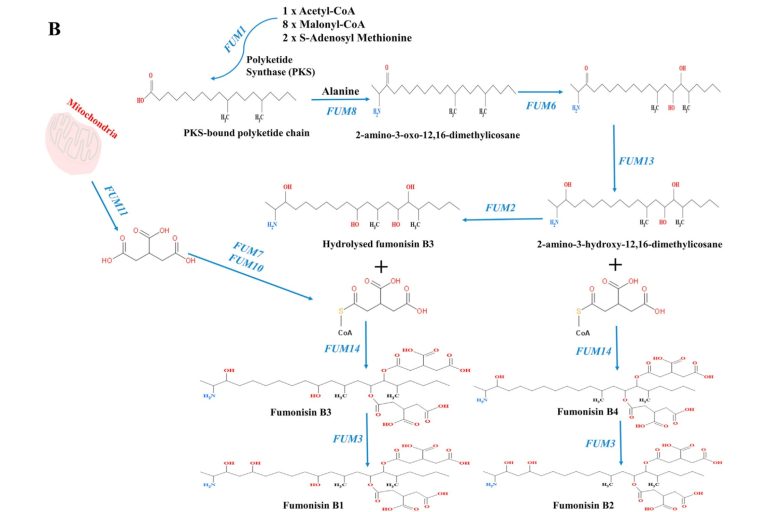
Figure 3. Proposed pathways for fumonisin biosynthesis (Butchko et al., 2006; Seo et al., 2001)
Biosynthesis Regulation
The production of fumonisins by different strains of Fusarium verticillioides is highly variable. In addition to environmental conditions, factors such as the availability of water and nutrients also influence the synthesis of fumonisin. There are also genes located outside the FUM locus that are involved at different levels within the cell signaling pathways. These genes play a key role in activating genes at the FUM locus, thereby regulating fumonisin production (Fig 4).
The influence of environmental conditions:
The production of fumonisins is influenced by several environmental and nutritional factors. The acidic pH favors the synthesis of the toxin, while the alkaline pH inhibits it. The PAC1 gene involved in the pH response acts as a repressor. The nitrogen source also impacts production, with ammonium being an inhibitor, and the AREA gene upregulates fumonisin synthesis.
The carbohydrates present in corn affect the production of mycotoxins, especially when the fungus grows on amylopectin or dextrin. The HXK1 gene, which perceives sugars, is responsible of synthesis regulation, with an 80% decrease in its absence.
Carbohydrate transport, regulated by the FST1 gene, is also key to fumonisins production. The transcriptional factors Zfr1 and Sda1 positively and negatively regulate synthesis, respectively. In addition, the FUM21 gene also has a positive role in the production of the toxins.
In summary, fumonisins synthesis is modulated by environmental (such as pH) and nutritional factors (such as nitrogen and carbohydrates sources), as well as gens and transcriptional factors that act positively or negatively (De la Torre-Hernández et al., 2014).
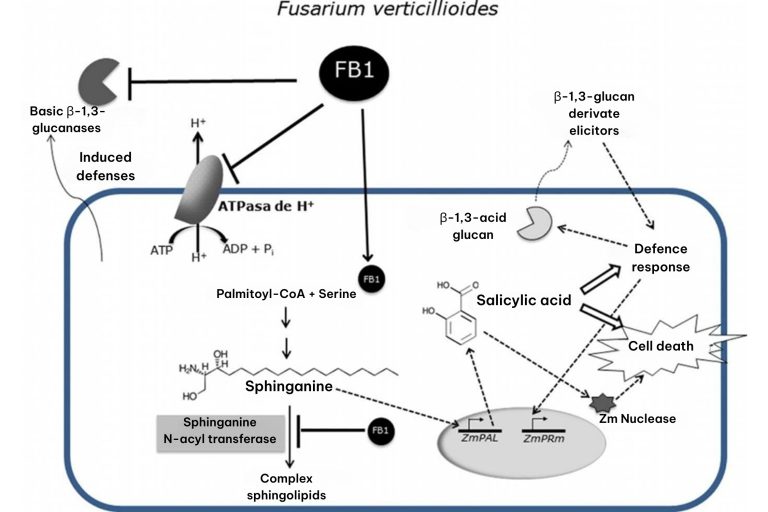
Figure 4. Regulation of fumonisin synthesis. Indicating positive and negative regulators of the FUM genes and location in the cell. Solid arrows indicate a positive effect while truncated lines indicate a negative effect (De la Torre-Hernández et al., 2014).
The production of fumonisin B1 (FB1) by Fusarium verticillioides is closely related to virulence, demonstrating that its synthesis occurs from the early stages of infection, even during the endophytic phase (Williams, L. D et al., 2006).
Fumonisins cause phytotoxic effects, such as reducing root and stem growth in corn seedlings. They also inhibit stem growth in plants. (Williams, L. D et al., 2007)
The production capacity of fumonisins increases the potential to infect corn seedlings, making the fungi more virulent. Therefore, the synthesis of fumonisins play a crucial role in fungi virulence during infection of corn seedlings, but they are not necessary for the infection or rot of stems and cobs. Evidence suggests that although fumonisins contribute to colonization in the early stages of infection, their impact decreases in later stages of the disease. Also, due to their chemical stability, they can persist even after the fungus dies.
Molecular interactions of fumonisins
Fumonisin B1 (FB1) acts by inhibiting several molecular targets in plant cells, which contributes to its virulence in Fusarium verticillioides infecting cultures.
The main molecular targets affected are detailed below:
- Sphingganin N-acyl transferase: FB1 inhibits this enzyme, which is critical in sphingolipid biosynthesis, causing the accumulation of sphinganine and phytosphingosine. This accumulation alters the cell membrane and activates signaling pathways related to cell death. In corn, a greater accumulation of sphingganin and phytosphingosine is observed, which is related to necrotic lesions in leaves and cell death, favoring the fungus as a as a saprophyte (Merrill et al., 1993).
- Plasma membrane H+ ATPase: This enzyme is crucial for the maintenance of pH and membrane potential, essential for tissue elongation. FB1 inhibits ATPase H+, which reduces root elongation and acidification of the medium, contributing to the inhibition of plant growth (Liu, J et al., 2009).
- β-1,3-glucanases: These enzymes, involved in the defense of plants against fungi, are modulated by FB1. The toxin inhibits the activity of basic β-1,3-glucanases (Zitomer N.C. et al., 2010), while inducing the activity of an acidic glucanase, possibly mediated by salicylic acid (de la Torre-Hernández, et al. 2010) suggesting an alteration in the plant’s defense response. (Sánchez-Rangel, D., et al. 2012)
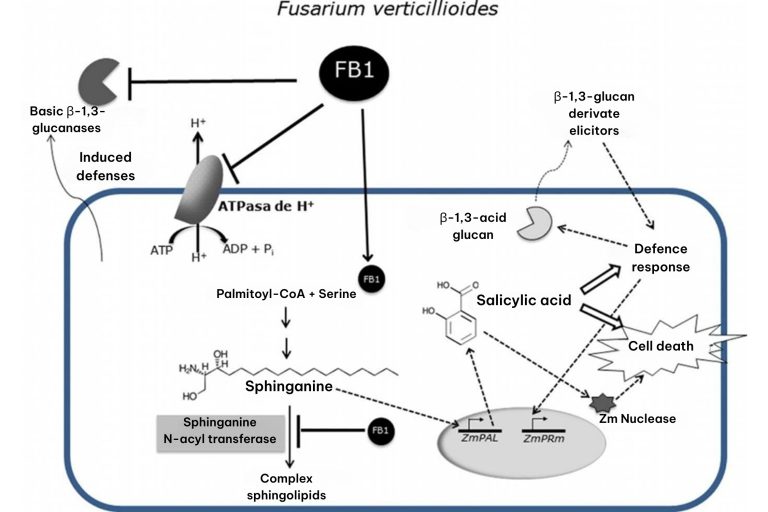
Figure 5. Inhibitory effects of fumonisin FB1 in plant cells on H+ ATPase, N-acyl transferase sphingamine and basic β-1,3-glucanases (De la Torre-Hernández et al., 2014).
Together, these molecular effects contribute to the damage and virulence of Fusarium verticillioides in maize, favoring the colonization of the fungus in plant tissues.
Conclusions
The synthesis of fumonisins, such as fumonisin B1 (FB1), is a key virulence factor for Fusarium. FB1 inhibits the biosynthesis of sphingolipids, altering the balance of cellular metabolites and causing cell death, which favors the proliferation of fungus in plant tissues and allows them to adopt parasitic behaviors.
In addition, FB1 is able to inhibit various plant defensive responses of the plant, which facilitates colonization and infection, and allows the fungus to damage crops more efficiently. This not only produces significant economic losses, but, when accumulated in grains and cereals, represents a toxicological risk for the animals that feed on them. Understanding the mechanisms that regulate their colonization and expression is crucial to optimizing crop management and minimizing harmful effects in the food chain.